“DIY Electrocharged Face Masks: Replicating N95 Filtration Using Household Items and Innovative Electrospinning Techniques”
Hello textile enthusiasts and tech innovators! As Textile Topher, I’m thrilled to share with you a groundbreaking approach to creating an N95-equivalent face mask, utilizing common household materials and a pinch of ingenuity. While face masks have become a staple in our fight against COVID-19, replicating the sophisticated filtration capabilities of an N95 mask seemed a daunting challenge—until now. Innovative research from the Nonlinear and Non-equilibrium Physics Unit at OIST Graduate University, led by Mahesh M. Bandi, details an accessible method to craft your own electrocharged filtration mask. Let’s dive deep into this fascinating world and reveal the secrets behind their inventive technique!
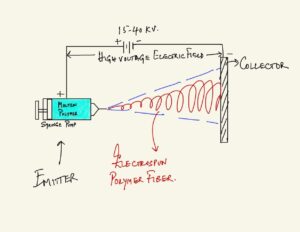
Understanding the N95 Filtration Principle
First, let’s break down what makes the N95 masks so effective. N95 masks filter air using three primary mechanisms: inertial impaction, diffusion, and electrostatic attraction. Together, these principles work to trap particulates from aerosols to virions:
1. Inertial Impaction**: Particles larger than 1 micron, due to their inertia, slam into the mask fibers instead of flowing around them.
2. Diffusion**: Smaller particles (below 1 micron) undergo Brownian motion, colliding with fibers and sticking to them.
3. Electrostatic Attraction**: This is the magic component. Electrocharged layers in N95 masks attract and trap oppositely charged particles, regardless of their size.
It’s this third principle, the electrostatic attraction, that truly sets N95 masks apart from homemade or standard commercial masks.
Electrocharged Filtration Explained
We’ve all experienced triboelectric charging—static electricity that comes from rubbing fabrics together. Fabric like wool or synthetic materials such as nylon accumulate static charge through friction. Leveraging this principle, researchers have used electrospinning to produce charged fibers essential for sophisticated filtration.
Electrospinning** is a process where viscous molten polymer is ejected through tiny orifices under a high-voltage electric field. The polymer jets out as fine nanofibers, depositing onto a grounded collector—a delicate dance of physics and material science.
While industrial electrospinning setups are complex, our resourceful researchers have found a way to mimic this process using a common household device—a cotton candy machine!
Crafting the Electrospun Polymer Fabric at Home
Here’s where it gets delightfully DIY. By modifying a regular cotton candy machine, we can achieve an effective, high-throughput electrospinning process at home:
1. Modified Emitter and Collector Setup**: The central spinning container (emitter) in the cotton candy machine, usually reserved for sugar, is now utilized to melt polypropylene (a common plastic with a melting point of about 160°C—perfect for cotton candy machines).
2. Generating Electrospun Fibers**: Replace sugar with powdered polypropylene (easily made by pulverizing plastic bottles) and melt them in your emitter. Spinning at high speeds, the centrifugal force shoots out the molten polymer, which forms fine nanofibers as it cools and collects on the drum surface.
3. Creating an Electrostatic Field**: Although traditional electrospinning uses a high-voltage DC field, our method uses mechanical acceleration via the machine’s centrifugal force. Supplementing this with a modest DC electric field (12-24V) encourages the fibers to gain and maintain electrostatic charges.
4. Safety and Sterility**: Ensure your plastic is free from contaminants by sterilizing it in a pressure cooker before use.
Thus, this adapted cotton candy method pioneers a home-spun version of creating electrospun nanofibers—a brilliant example of repurposing everyday items for high-tech solutions.
Detailed Characterization and Performance
Curious about how these homemade electrospun fabrics stack up against commercial N95 layers? Let’s delve into some meticulous characterizations:
1. Scanning Electron Microscope (SEM) Analysis**: SEM images of the home-produced fibers showed structures closely mirroring those of commercial N95 mask layers—from vast fabric expanses down to detailed nanoscopic features. Visually, they were virtually indistinguishable.
2. Particle Filtration Efficiency**: The performance was validated by testing the material’s ability to trap 50-nanometer fluorescent colloidal particles under varying airflows. Given the COVID-19 virion’s size (50-200 nm), these fibers showed promising protective potential.
Mask Construction and Practical Use
With the electrocharged polymer fabric in hand, let’s discuss constructing a functional mask:
1. Layering Technique**: Researchers recommend a structure of five layers—three layers of electrospun fiber sandwiched between two layers made from fibers spun without an electric field. This design maximizes filtration while maintaining breathability.
2. Assembly**: Cut the layered fabric into squares, fold, and stitch for a snug design. There’s also an accessible non-stitch method available from the New York Times by Farhad Manjoo, which can be adapted for use with this fabric.
Limitations and Considerations
Despite promising results, our homemade masks fall short in several areas compared to certified N95 masks:
1. Fit and Seal**: N95 masks are intricately designed to prevent air leakage around the edges, whereas our design may have gaps.
2. Humidity Resistance**: The electrocharges degrade within hours under humid conditions (like breathing), limiting the mask’s effective lifespan.
3. Certifications and Safety**: This mask isn’t tested or certified by regulatory bodies like NIOSH. It’s crucial that users understand these are improvisational measures and not substitutes for certified PPE.
Conclusion and Acknowledgments
In conclusion, while not a silver bullet, this novel mask design presents an accessible, effective interim solution, especially where professional-grade masks are scarce. Hats off to the determined researchers at OIST Graduate University for their innovative approach and for sharing their work freely.
Acknowledgments go to Ms. Noriko Ishizu and the Nanofab team at OIST for SEM support, the Imaging Section for confocal microscopy assistance, and the OIST Digital Services Section for their swift webpage updates. Their collective effort brings this accessible tech into the public realm.
Ready to embrace your inner engineer? With curiosity, a cotton candy machine, and a bit of DIY spirit, you too can transform common materials into high-tech protective gear—proving that ingenuity is the sharpest tool in a crisis. Until next time, stay safe and let your textile talents shine!
Keywords: Electrostatic, Polymer, Electrospinning, (Post number: 204), Nanofibers, Filtration